HYDROGEN BASIC CONcept
Hydrogen is the lightest and most abundant element in the universe. In its pure form (H2), it is a colourless and odourless gas.
However, since it combines easily with other elements, hydrogen is rarely found by itself in nature and is usually found as a part of other compounds, including fossil fuels, plant material, and water.
Hydrogen is an energy carrier, not an energy source, meaning that it stores and delivers energy in a usable form. Like electricity, the most familiar energy carrier, it can be generated from a wide range of sources.
Hydrogen Production
Hydrogen can be produced using a variety of domestic energy resources -fossil fuels, such as coal and natural gas, with carbon capture and sequestration; renewables, such as biomass; electricity from renewable energy technologies, including solar, wind, geothermal, and hydropower; and thermal energy or electric power from nuclear.
Hydrogen Delivery
Since it can be produced from several sources and using various methods, hydrogen can be produced at large production plants and transported to users. It can also be produced locally, using small generators, possibly at refuelling stations, eliminating the need for long-distance transport. Hydrogen is currently transported by road via cylinders, tube trailers, cryogenic tankers, and in pipelines. However, hydrogen pipelines currently only exist in a few regions of the United States. The delivery infrastructure for hydrogen will require high-pressure compressors for gaseous hydrogen and liquefaction for cryogenic hydrogen. Both currently have significant capital and operating costs, and energy inefficiencies.
Hydrogen Storage
Finding a cost-effective method of storing hydrogen on a vehicle is a challenge. While hydrogen contains more energy per weight than any other energy carrier, it contains much less energy by volume. Hydrogen may be stored as a liquid, a high-pressure gas, or by chemical methods; all are under intense development.
CHALLENGES
Weight and Volume
The weight and volume of hydrogen storage systems are presently too high, resulting in inadequate vehicle range. Materials and components are needed that allow compact, lightweight, hydrogen storage systems while enabling greater than 300-mile range in all light-duty vehicle platforms.
Efficiency
Energy efficiency is a challenge for all hydrogen storage approaches. The energy required to get hydrogen in and out is an issue for reversible solid-state materials. Life-cycle energy efficiency is a challenge for chemical hydride storage in which the by-product is regenerated off-board. In addition, the energy associated with compression and liquefaction must be considered for compressed and liquid hydrogen technologies.
Cost
The cost of on-board hydrogen storage systems is high, particularly in comparison with conventional storage systems for petroleum fuels. Low-cost materials and components for hydrogen storage systems are needed, as well as low-cost, high-volume manufacturing methods.
Codes and Standards
Applicable codes and standards for hydrogen storage systems and interface technologies, which will facilitate implementation/commercialization and assure safety and public acceptance, are being established. Standardized hardware, operating procedures, and applicable codes and standards, are required.
POTENTIAL APPLICATIONS
Most of the hydrogen produced is used as a chemical, rather than a fuel, in a variety of commercial applications:
- Commercial fixation of nitrogen from the air to produce ammonia for fertilizer (about two-thirds of commercial hydrogen is used for this)
- Hydrogenation of fats and oils, in which vegetable oils are changed from liquids to solids; shortening is an example of a hydrogenated oil
- Methanol production, in hydrodealkylation, hydrocracking, and hydrodesulphurization
- Welding
- Hydrochloric acid production
- Metallic ore reduction
- Cryogenics and the study of superconductivity (liquid hydrogen)
Hydrogen’s main use as a fuel is in the space program. Today hydrogen fuels both the main engine of the Space Shuttle and the onboard fuel cells that provide the Shuttle’s electric power.
Hydrogen can be used in fuel cells to power a wide variety of applications, both mobile and stationary, small- and large-scale. Fuel cells can be used to provide clean energy for transportation. And because they are modular, fuel cells can provide heat and electricity for single homes or to supply the energy to run an entire large commercial building, to provide a small amount of electricity to a community grid, or a large amount of electricity to a large grid network.
Hydrogen can be used to generate electricity for our homes and office buildings, through the use of gas turbines and microturbines (small gas turbines). Conventional gas turbines can be modified to run efficiently on hydrogen or hydrogen/natural gas blends. Microturbines can provide high-efficiency reliable power for smaller-scale applications.
Hydrogen can also be used in internal combustion engines for both stationary and mobile applications, powering industrial processes, ocean fleets, cars, and buses. As with gas turbines, conventional combustion engines can be modified to run efficiently on hydrogen or hydrogen/natural gas mixtures for these applications.

HYDROGEN PROPERTIES
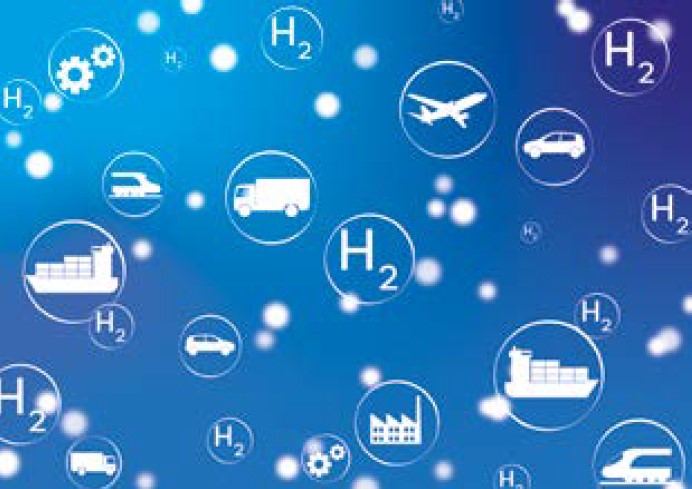
Hydrogen is a colourless, odourless, tasteless, and non-poisonous gas under normal conditions on Earth. It typically exists as a diatomic molecule, meaning each molecule has two atoms of hydrogen; this is why pure hydrogen is commonly expressed as “H2“.
Hydrogen is the most abundant element in the universe, accounting for 90 percent of the universe by weight. However, it is not commonly found in its pure form, since it readily combines with other elements. It is also the lightest element, having a density of 0.08988 grams per liter at standard pressure.
Hydrogen has several important chemical properties that affect its use as a fuel:
It combines with oxygen to form water, which is absolutely necessary for life on this planet.
It has a high energy content per weight (nearly 3 times as much as gasoline), but the energy density per volume is quite low at standard temperature and pressure. Volumetric energy density can be increased by storing the hydrogen under increased pressure or storing it at extremely low temperatures as a liquid. Hydrogen can also be adsorbed into metal hydrides.
Hydrogen is highly flammable; it only takes a small amount of energy to ignite it and make it burn. It also has a wide flammability range, meaning it can burn when it makes up 4 to 74 percent of the air by volume.
Hydrogen burns with a pale-blue, almost-invisible flame, making hydrogen fires difficult to see.
The combustion of hydrogen does not produce carbon dioxide (CO2), particulate, or sulphur emissions. It can produce nitrous oxide (NOX) emissions under some conditions.
Hydrogen can be produced from renewable resources, such as by reforming ethanol (this process emits some carbon dioxide) and by the electrolysis of water (electrolysis is very expensive).
Sources: Adapted from US DOE
To see more : Video – properties of hydrogen – https://www.youtube.com/watch?v=U-MNKK20Z_g
THE COLORS OF HYDROGEN

Green hydrogen
Green hydrogen is produced through water electrolysis process by employing renewable electricity. The reason it is called green is that there is no CO2 emission during the production process. Water electrolysis is a process which uses electricity to decompose water into hydrogen gas and oxygen.
Blue hydrogen
Blue hydrogen is sourced from fossil fuel. However, the CO2 is captured and stored underground (carbon sequestration). Companies are also trying to utilise the captured carbon called carbon capture, storage and utilisation (CCSU). Utilisation is not essential to qualify for blue hydrogen. As no CO2 is emitted, so the blue hydrogen production process is categorised as carbon neutral.
Grey hydrogen
Grey hydrogen is produced from fossil fuel and commonly uses steam methane reforming (SMR) method. During this process, CO2 is produced and eventually released to the atmosphere, which make these hydrogen technology unsuitable for a route towards net-zero emissions.
Black or brown hydrogen
Black or brown hydrogen is produced from coal. The black and brown colours refer to the type bituminous (black) and lignite (brown) coal. The gasification of coal is a method used to produce hydrogen. However, it is a very polluting process, and CO2 and carbon monoxide are produced as by-products and released to the atmosphere.
Turquoise hydrogen
Turquoise hydrogen can be extracted by using the thermal splitting of methane via methane pyrolysis. The process, though at the experimental stage, remove the carbon in a solid form instead of CO2 gas.
Purple hydrogen
Purple hydrogen is made though using nuclear power and heat through combined chemo thermal electrolysis splitting of water.
Pink hydrogen
Pink hydrogen is generated through electrolysis of water by using electricity from a nuclear power plant.
Red hydrogen
Red hydrogen is produced through the high-temperature catalytic splitting of water using nuclear power thermal as an energy source.
White hydrogen
White hydrogen refers to naturally occurring geological hydrogen, fund in underground deposits and create trough fracking – there are no strategies to exploit this hydrogen at present.
Hydrogen itself is a colourless gas but there are around nine colour codes to identify hydrogen. The colours codes of hydrogen refer to the source or the process used to make hydrogen. These codes are: green, blue, grey, brown or black, turquoise, purple, pink, red and white.
During early stages of the energy transition, the use of Blue Hydrogen could facilitate the growth of a hydrogen market. Around three-quarters of hydrogen is currently produced from natural gas. Retrofitting with CCS would allow the continued use of existing assets while still achieving lower GHG emissions. This is an option is to produce hydrogen with lower GHG emissions while reducing pressure on the renewable energy capacity installation rate to produce green hydrogen. Notably, industrial processes like steel production may require a continuous flow of hydrogen; blue hydrogen could be an initial solution while green hydrogen ramps up production and storage capacity to meet the continuous flow requirement. However, blue hydrogen has limitations that have so far restricted its deployment: it uses finite resources, is exposed to fossil fuel price fluctuations, and does not support the goals of energy security. Moreover, blue hydrogen faces social acceptance issues, as it is associated with additional costs for CO2 transport and storage and requires monitoring of stored CO2. In addition, CCS capture efficiencies are expected to reach 85-95% at best, which means that 5-15% of the CO2 will still be emitted. And these high capture rates have yet to be achieved. In sum, the carbon emissions from hydrogen generation could be reduced by CCS but not eliminated. Moreover, these processes use methane, which brings leakages upstream, and methane is a much more potent GHG per molecule than CO2. This means that while blue hydrogen could reduce CO2 emissions, it does not meet the requirements of a net-zero future. For these reasons, blue hydrogen should be seen only as a short-term transition to facilitate the uptake of green hydrogen on the path to net-zero emissions.